Our paper entitled “Cerebellar neurodynamics predict decision timing and outcome on single-trial level” has been published in Cell.
Decision making and motor planning are key cognitive functions that underly all goal-directed actions performed by animals. These cognitive functions are thought to depend on interactions between different, separated brain regions. However, how brain-wide dynamics of neuroactivity leads to action selection is less understood. Moreover, a hallmark of decision making, and more generally all animal behavior, is its variability across trials For seemingly random reasons, animals sometimes select different actions despite all observable inputs being the same. This variability points to changes of the underlying functional connectivity which typically cannot be captured at the trial-by-trial level by most neurotechnologies nor by static and trial-averaged tuning frameworks for representation of motor responses.
In this work we have investigated the neuronal basis of motor planning and decision making at the single-trial level, at single-cell resolution and on the level of the whole brain. We have demonstrated that decision making relies on integration and coordination of information across different brain regions. Within the brain-wide network, we discovered a particularly strong preparatory activity in the cerebellum which allowed us to quantitatively predict both, the time point and the outcome of the decisions up to ~10 seconds in advance of the animal’s behavior on a trial-by-trial basis.
To investigate the neural basis of motor planning, we have combined high-speed volumetric calcium imaging based on light-field microscopy (LFM) – as previously developed in our lab – with a new operant conditioning learning paradigm in larval zebrafish, Relief Of Aversive Stimulus by Turn (ROAST) in which fish learn to make a targeted tail movement in a specific direction (left or right) to obtain relief from a heat stimulus (Figure 1A, B).
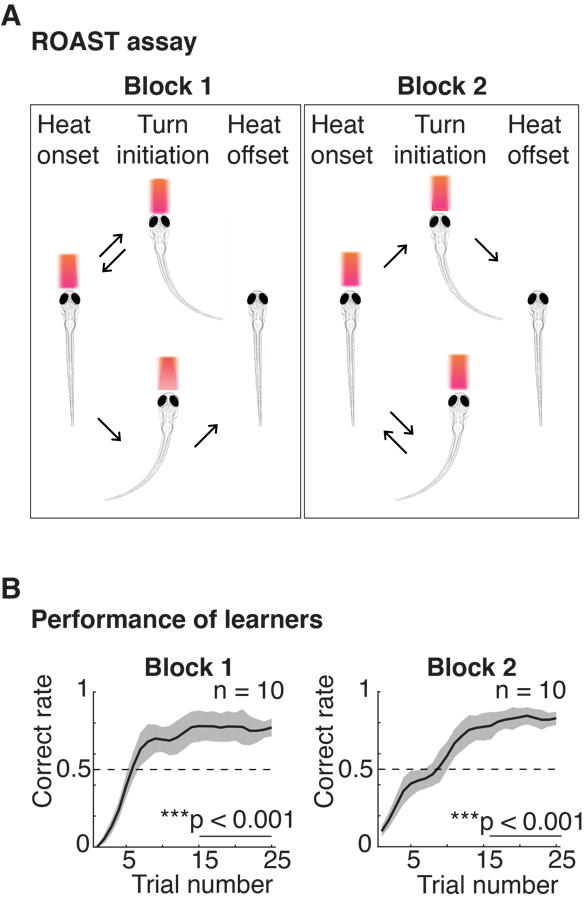
Figure 1 | An operant conditioning assay in larval zebrafish. (A) Relief of Aversive Stimulus by Turn (ROAST) in head-fixed larval zebrafish. Block 1: reward direction is set to be tail movement to the left, block 2: reward direction is set to be a tail movement to the right (B) Averaged performance as a function of trial number from 7-9 dpf larvae (n = 10) (mean ± SEM). The correct rate in the last 10 trials is significantly above 0.5, ***p < 0.001 for both blocks.
Using LFM, we recorded brain-wide neuroactivity at a volumetric frame rate of 10 Hz from a volume of 700 × 700 × 200 μm3, with all voxels being acquired truly simultaneously. Using volumetric reconstruction of the LFM data, followed by neuronal signal extraction, we obtained timeseries from ~5,000 of the most active neurons across the brain (Figure 2A). By applying dimensionality reduction techniques to the brain-wide neuronal timeseries, we found global, recurring dynamics of brain states that exhibit pre-motor bifurcations towards mutually exclusive decision outcomes, well before turn initiations at the single-trial level (Figure 2B), despite different decision times.
Figure 3 | Preparatory activity in the cerebellum is highly predictive of decision outcome. (A) Mapping of functional neuronal t-SNE clusters onto the zebrafish brain anatomy reveals distinct overlap with specific anatomical regions. (B) Ipsilateral cerebellum displays strong pre-motor ramping activity in correct trials. Data are averaged over trials. Neurons that display a strong post-turn activity in incorrect trials also have an early onset time and a strong pre-motor activity in correct trials. (C) Contralateral cerebellum shows little pre-motor activity. (D) Top demixed principal components (dPCs) from each functional neuronal cluster (see main text).
These global dynamics arise from a distributed network (Figure 3A), which displays trial-by-trial changes in the functional connectivity that are causally that are causally linked to the decision outcomes.
Within this brain-wide network, we discovered that the ipsilateral – with respect to the reward direction – cerebellum shows a particularly strong pre-motor activity during the correct trials. This was in contrast to what we observed for the contralateral cerebellum and during incorrect trials (Figure 3B, C). This discovery in the cerebellum was made by searching for the neural activity within each functional cluster that contributed to the observed pre-motor bifurcation of brain states and thus would be predictive of the motor response, using demixed principal component analysis (dPCA) (Figure 3D). dPCA decomposes the activity of a neuronal population into a few task-related components, such as different stimuli and decisions. We found the dPC1 of all clusters to account for at least ~50% of the observed variance in the corresponding cluster, and to exhibit a decision-independent response. dPC1 of Cluster 1 showed increased activity only after heat onset. dPC1 of Cluster 2 (contralateral ARTR) showed increased activity only after turn initiation, suggesting it is encoding for movements, while dPC1 of Cluster 3 (habenula) and Cluster 4 (ipsilateral cerebellum and ARTR) displayed a linear increase in activity upon heat onset followed by a further increase and a secondary peak after turn initiation, suggesting their potential involvement in sensorimotor transformation. dPC1 of Cluster 5 (contralateral cerebellum) and Cluster 6 (thalamus) showed an overall decreased activity upon heat onset (in contrast to the neuroactivity in Cluster 4 and Cluster 1, respectively), followed by an increase of activity and a secondary peak after turn initiation. dPC2 explained ~25% of observed variance in each cluster. Importantly, we found that dPC2 exhibited in all clusters a decision-dependent behavior with a clear separation of correct versus incorrect decisions, in some clusters prior to and in some clusters after initiation of motor behavior. Among these clusters, Cluster 4, the ipsilateral cerebellum and ARTR, allowed for a reliable decoding of the turn direction well before turn initiation.

Figure 3 | Preparatory activity in the cerebellum is highly predictive of decision outcome. (A) Mapping of functional neuronal t-SNE clusters onto the zebrafish brain anatomy reveals distinct overlap with specific anatomical regions. (B) Ipsilateral cerebellum displays strong pre-motor ramping activity in correct trials. Data are averaged over trials. Neurons that display a strong post-turn activity in incorrect trials also have an early onset time and a strong pre-motor activity in correct trials. (C) Contralateral cerebellum shows little pre-motor activity. (D) Top demixed principal components (dPCs) from each functional neuronal cluster (see main text).
By analyzing the joint bilateral neuronal activity of the cerebellum, we developed a competition-cooperation framework, in which turn directions are determined by the difference in neuroactivity between the ipsilateral and contralateral hemispheres (Figure 4). and the rate of bi-hemispheric population ramping activity predicts the decision time (Figure 5). We confirmed this causal relationship between the neural activity and behaviors, by lesion in the ipsilateral and/or contralateral hemispheres of the cerebellum.

Figure 4 | Ipsi-contra competition in the cerebellum determines decision outcome. (A) Trial-by-trial comparison of pre-motor neuronal activity between ipsilateral and contralateral sides for correct versus incorrect trials, data pooled across 5 learner fish. In the 144 correct trials from 5 learners, the ipsilateral pre-motor activity (median > 0) is significantly higher than the contralateral side (***p < 0.001), while in the 56 incorrect trials, the ipsilateral pre-motor activity (median < 0) is significantly lower than the contralateral side (***p < 0.001, left panel). Wilcoxon signed rank test, one-tailed. (B) Decision direction can be predicted with an average accuracy of 80% and as early as 10 seconds in advance.

Figure 5 | Bilateral cooperation of cerebellar neuroactivity determines decision time. (A) Monotonic, quasi-linear ramping activity from bilateral cerebellar population, grouped by different decision time. (B) Ramping rate of bilateral cerebellar population activity is inversely correlated with the decision time at the single-trial level across multiple animals (color-coded). (C) The accuracy of the predicted time of movement initiations increases with time. Predicted time is 22.3 s ± 2.5 s when t = 20 s, mean ± SEM.
Therefore, the pre-motor activity in the cerebellum was predictive of both, the turn direction and the specific time point at which the fish would execute the turn in each trial, more than 10 seconds before movement initiation (Movie 2).
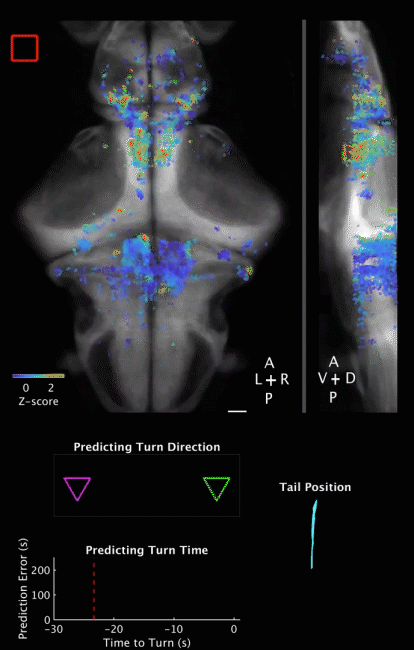
Movie 2 | Predicting decision outcome and time from cerebellar neuronal activity at the single-trial level. Movie of calcium imaging data during a ROAST trial, together with temporal evolution of prediction for both decision outcome and decision time. Prediction is made from the cerebellar neuronal activity at the single trial level. The example is a correct trial, with the training direction towards the right side. Movie starts 5 s before heat onset and ends 5 s after movement initiation. The prediction is evaluated starting at heat onset and updated until movement initiation.
Top panels: Maximum projections of neuron centroids, superimposed on an anatomical reference, Tg(elavl3:H2B-RFP) from the Z-Brain Atlas (Randlett et al., 2015). Filled red square indicates Heat ON, hollow red square indicates Heat OFF. Scale bar, 50 µm.
Middle panel: Outcome of a binary prediction for turn direction. Hollow triangles indicate the possible outcomes (left versus right), and filled triangles indicate predicted outcome, color coded green for the right (correct) side and magenta for the left (incorrect) side.
Bottom left panel: Evolution of error in predicted turn time, i.e. the difference between the prediction and the actual turn time. Red dashed line indicates heat onset; white dashed line indicates turn initiation.
Bottom right panel: Plot of tracked tail segments (n = 9 segments). Each rendered frame is the maximum projection of 16 consecutive recorded frames, to match behavioral recording frame rate (160 fps) to brain imaging frame rate (10 Hz). Playback is in real time (10 fps).
To determine which of the main cerebellar cell types contributed primarily to ramping activity, we used various cell-type-specific transgenic lines and revealed the granular cell population as the main contributing cell type. Based on our observations, we provide a cerebellar model for how multimodal information is transformed into decision signals (Figure 6): During the pre-motor period, the multimodal input to the cerebellum could be amplified by the divergent mapping of the mossy fiber input to the granular cells and then integrated by the eurydendroid neurons and fed into the ARTR, i.e. the hindbrain oscillator. After motor response, two feedback signals are sent: The inferior olive activates the Purkinje cells via climbing fibers on the contralateral side of the cerebellum, while a self-excitatory ipsilateral feedback is sent to the ARTR. The signal to the contralateral Purkinje cells can serve as an error signal that, together with inputs from other brain areas, facilitates synaptic plasticity.
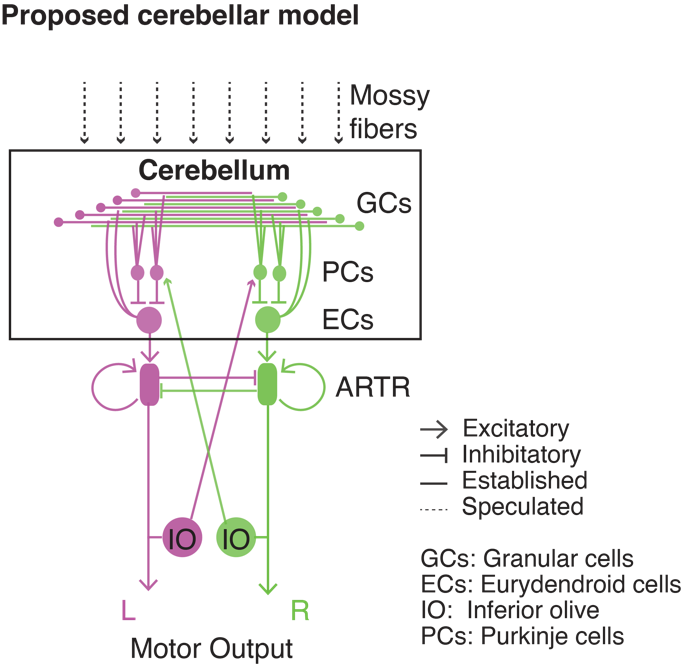
Figure 6 | Proposed model for decision making network in cerebellum. Schematic overview of the proposed neurocircuitry underlying motor planning and action selection in our task: The cerebellum integrates multimodal information, as well as other sensory and motor signals from various precerebellar nuclei, via the mossy fibers to perform action selection and movement preparation and generation. The cerebellar dynamics we have described are relayed to the ARTR via the eurydendroid cells, which initiate a motor command that is sent to the spinal cord. Following movement initiation, the cerebellum receives feedback from the inferior olive, thereby forming a closed.
In summary, in this study we identify the neuronal basis of motor planning and decision making at the single-trial level, at single-cell resolution and the level of the whole brain. Our results extend the traditional view on the role of the cerebellum in motor control and provide evidence that points toward a cognitive role of the cerebellum and its significance for motor planning.
Relevant publication:
Qian Lin, Jason Manley, Magdalena Helmreich, Friederike Schlumm, Jennifer M. Li, Drew N. Robson, Florian Engert, Alexander Schier, Tobias Nöbauer, Alipasha Vaziri. Cerebellar neurodynamics predict decision timing and outcome on single-trial level. Cell 180, 1-16 (2020)
(Download)