It is widely accepted that that the patterns of action potentials in neuronal circuits underlie cognitive functions such as perception, memory and decision making. Thus, knowledge on structural connectivity in neuronal circuits is necessary for understanding information representation and processing in local circuits. However, as some examples of well-characterized neuronal architectures illustrate, structural connectivity alone it is not sufficient to predict how input stimuli are mapped onto activity patterns of neuronal populations and how the collective dynamics of all neurons in the network leads to behavior. Addressing this challenge has been hampered by lack of appropriate tools and methods that allow parallel and spatiotemporally specific application of excitation patterns onto neuronal populations while capturing the dynamics of the network activity at high spatial and temporal resolution. We are trying to address this issue by developing new optogenetics and imaging techniques based on a combination of advanced optical techniques, molecular biology and mathematical methods.
The recently discovered class of genetically expressible photoactivatable ion-channels such as Channelrhodopsin has enabled the optical control of neural activity. The most widely used approach has been the optical activation of the genetically expressed light-gated ion-channel, Channelrhodopsin-2 (ChR2), to initiate population activity in neuronal circuits. However, given the low channel conductance, the initiation of action potentials is only possible when a sufficiently large number of channels are activated at the same time, which has made single cell resolution of optogenetic activation a major challenge.
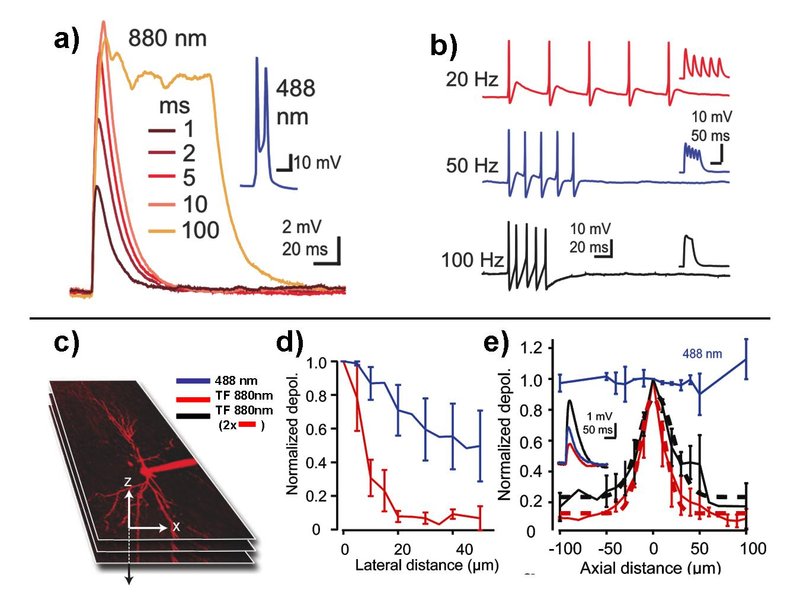
To overcome these limitations, we have recently developed a scheme for fast, selective and targeted control of neuronal activity with single cell resolution in mouse and rat hippocampal slices. Using the scanningless technique of temporal focusing for which the axial beam profile can be controlled independently of its lateral distribution, large number of channels on individual neurons can be excited simultaneously leading to strong depolarizations (Fig. 1).
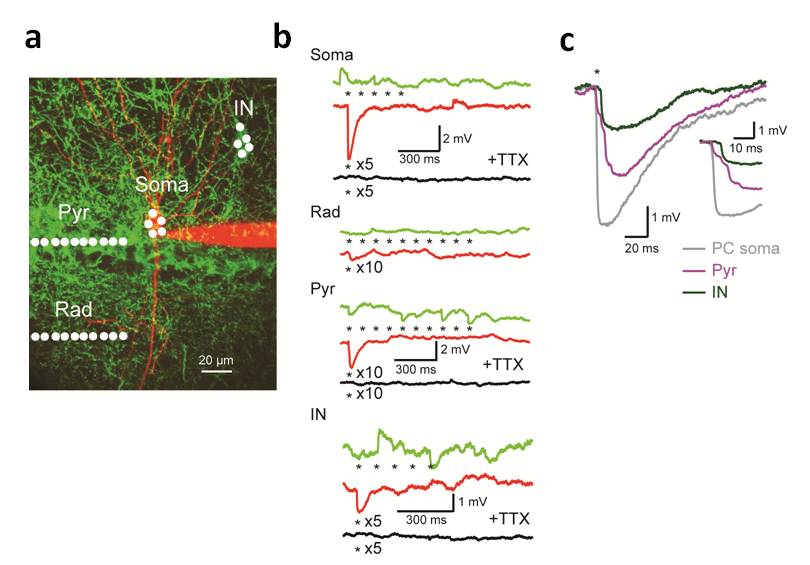
Additionally, using this approach cellular compartments such as dendrites and large inhibitory synaptic terminals can be activated selectively with high spatiotemporal precession (Fig. 2). This allows for fine manipulation of neuronal activity to study and control the function of neuronal microcircuits in vitro and in vivo. In particular in the context of neuronal circuit mapping and dendritic integration it can be used as a tool for high throughput mapping of connectivity and provides the means to study mechanism of neuronal input integration on a single neuron with unprecedented spatiotemporal resolution.
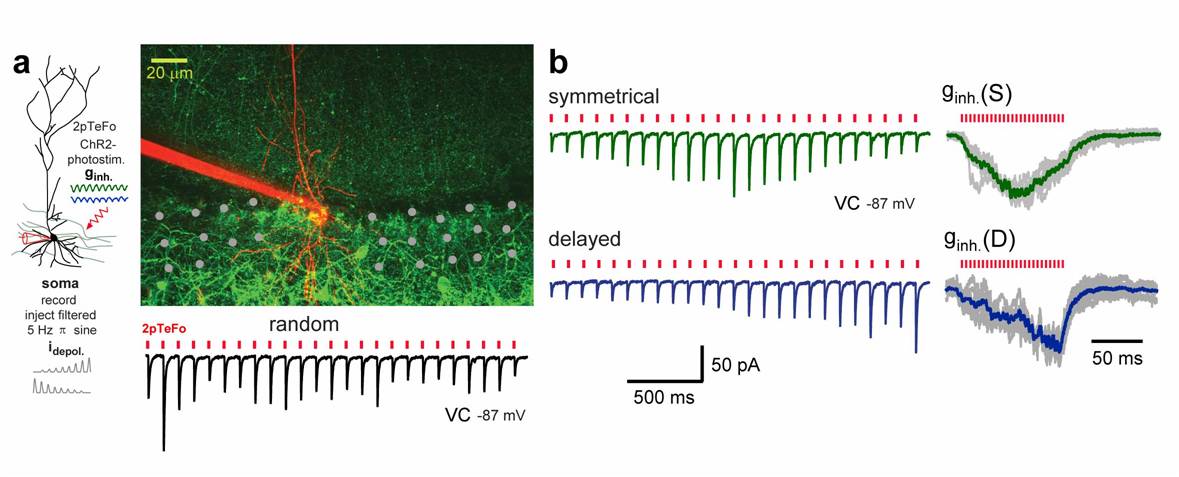
At the same time the application of this method on the neuronal network level allows to deliver defined neuronal input patterns onto neuronal populations. This can be used to interfere and manipulate the spatio-temporal structure of the pre-synaptic inputs that of a local neuronal population is receiving. We have demonstrated the power of this approach by mimicking the perisomatic inhibition patterns that hipocampal CA1 pyramidal neurons receive during place cell activity (see Fig. 3). We are interested in using the speed and flexibility that this technique provides to explore role of stochastically induced sub-threshold spontaneous activity pattern of individual, how they are integrated into the network dynamics by combing it with Ca2+ imaging and electrophysiology.
Relevant publications:
A. Vaziri and V. Emiliani,
Reshaping the Optical dimension in optogenetics
Current Opinion in Neurobiology 22, 128-137 (2012).
(Download)
Andrasfalvy, BK., Zemelman, BV., Tang, J., Vaziri, A.
Two-photon single-cell optogenetic control of neuronal activity by sculpted light
Proc. Natl. Acad. Sci. USA 107(26), 11981-6 (2010).
(Download)
Losonczy, A., Zemelman, BV., Vaziri, A., Magee, JC.
Network mechanisms of theta related neuronal activity in hippocampal CA1 pyramidal neurons
Nature Neuroscience 13(8), 967-72 (2010).
(Download)