Our paper entitled “Brain-wide 3D light-field imaging of neuronal activity with speckle-enhanced resolution” has been published in Optica.
A major challenge in neuroscience is to sample large-scale neuronal activity at high speed and resolution. While calcium (Ca2+) imaging allows high-resolution optical read-out of neuronal activity, it remains challenging to sample large-scale activity at high speed, as most available imaging microscopes provide a trade-off between speed and the size of the acquisition volume. One promising method that avoids the trade-off between the acquisition rate and volume size is light-field microscopy in which the full 3D profile of an object is imaged simultaneously, thereby offering high speed at the cost of reduced spatial resolution.
In our recent article in Optica, we introduce speckle light-field microscopy (“speckle LFM”), which utilizes speckle-based structured illumination to enhance spatial resolution of light-field microscopy. Using speckle LFM we demonstrate brain-wide recording of neuronal activity in larval zebrafish at 10 Hz volume rate and at 1.4 times higher resolution compared to conventional light-field microscopy (“linear LFM”) and with suppressed background fluorescence. In addition to improving resolution of spatial structure, we show that the increased resolution reduces spurious signal crosstalk between neighboring neurons.
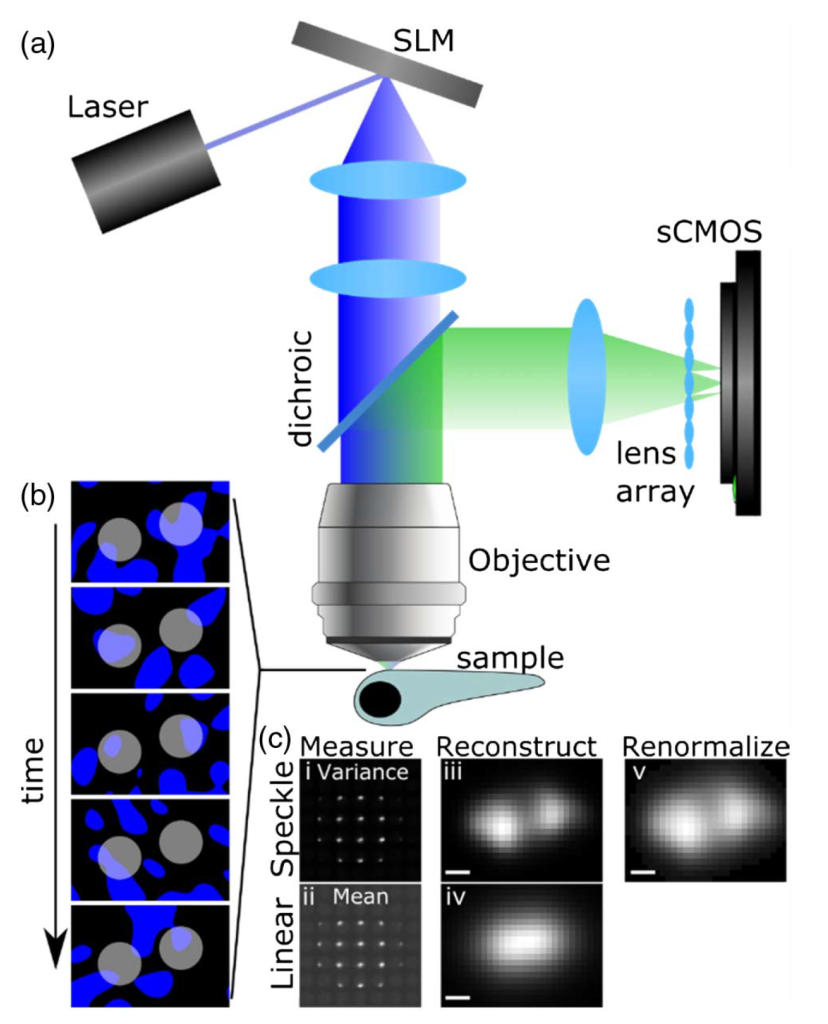
In speckle LFM, the sample is illuminated with a fluctuating sequence of speckle illuminations [Figs. 1(a) and 1(b)]. Speckle is a random intensity pattern that forms when a set of light wavefronts interfere; it can be produced easily by shining a laser beam onto a diffuser, or, as in our case, onto a programmable spatial light modulator device (SLM). The speckle pattern is characterized by an average speckle grain size, which in our case is limited by diffraction effects due to the finite numerical aperture of the microscope objective.
Fluorophores that are further separated than the speckle grain size experience statistically independent fluctuations of intensity, and thereby generate statistically independent fluctuations in their emitted fluorescence. These fluctuations encode an image of the object onto the variance of a recorded image sequence. Unlike the linear measurement that is based on the measured intensities, this variance image encodes nonlinear information about the sample, as it convolves the square of the fluorophore brightness with the square of the point-spread function (PSF). The nonlinearity in the imaging PSF provides higher resolution than the linear measurement while at the same time suppressing the background [Fig. 1(c)].
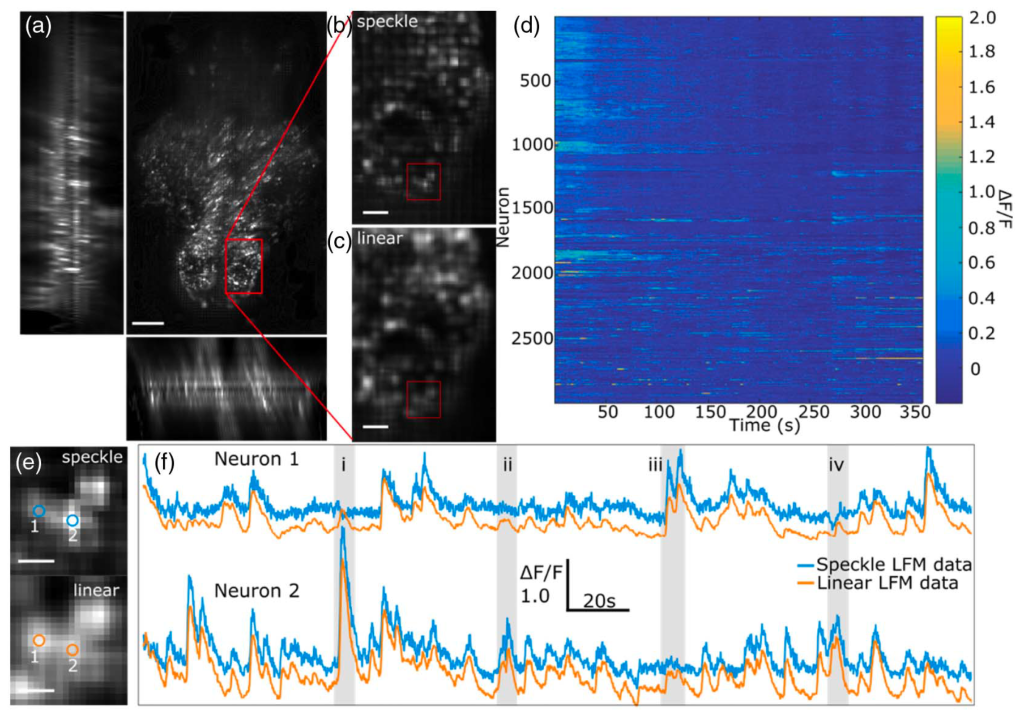
Using our speckle LFM we imaged neural activity at 10 Hz volume rate in 7-day old larval zebrafish expressing GCaMP6s. Whole-brain imaging of the fish was performed and the acquired images reconstructed as described above using both speckle LFM and linear LFM (Fig. 2). The improved resolution of speckle LFM was clearly visible, allowing visual identification of many bright loci that were not clearly distinguished with linear LFM [Figs. 2(b) and 2(c)].
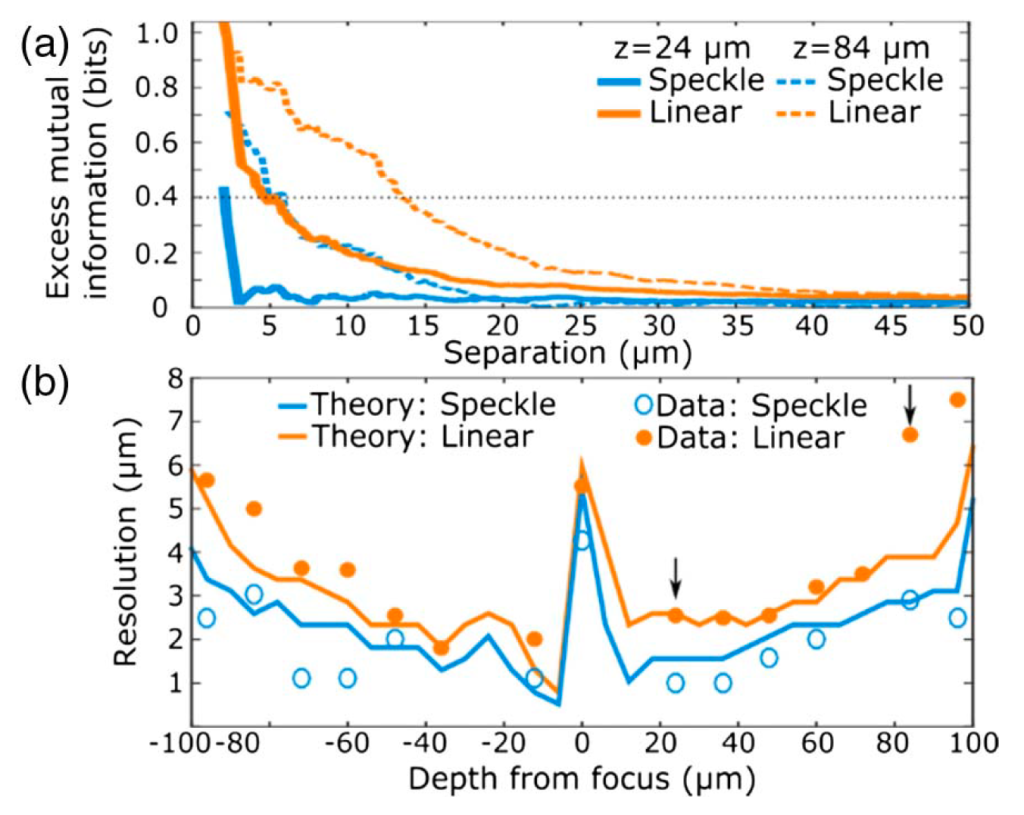
To quantify crosstalk between ROI pairs we evaluated the mutual information, which quantifies the degree of common signal in a pair of separate measurements. We found that the mutual information recorded in closely spaced ROIs was consistently higher in the linear LFM data than speckle LFM data, suggestive of measurement crosstalk arising from the limited spatial resolution of linear LFM. As shown in Fig. 3(a), the average mutual information is highest for ROIs that are closely separated, while at large separations there is no correlation between ROI separation and mutual information. The onset of this rise in mutual information was consistently observed at smaller separations with speckle LFM, indicating that measurement crosstalk rather than correlated activity dominated the observed rise in mutual information. Consequently, the onset of crosstalk allows quantification of the spatial resolution achieved in vivo.
The mutual information analysis was applied to estimate the resolution of both linear LFM and speckle LFM across the entire imaged volume. We found that speckle LFM achieved higher resolution than linear LFM at every recorded depth [Fig. 3(b)]. Use of speckle LFM improves resolution by a factor that varies with depth with a mean enhancement of 1.4 predicted theoretically, which agrees with the factor of 2.0±0.6 extracted from experimental data.
While our speckle LFM method is immediately applicable to functional imaging, we also note that the method has the potential to be dramatically improved with more elaborate approaches to 3D reconstruction. Speckle LFM also provides access to both the linear and nonlinear data, which could potentially be merged to profit from the relative advantages of the two methods, ideally combining the higher SNR of linear LFM with the higher resolution of speckle LFM. As such, we anticipate that speckle LFM could lead to exciting further advances in the future.
Relevant publication:
Michael A. Taylor, Tobias Nöbauer, Alejandro Pernia-Andrade, Friederike Schlumm, and Alipasha Vaziri,
Brain-wide 3D light-field imaging of neuronal activity with speckle-enhanced resolution
Optica 5, 345-353 (2018).
(Download)